Great Lakes Observing Systems
Great Lakes observing systems provide critical information to support natural resource decision making, public health protection, and navigation safety and efficiency.
With our partners at NOAA GLERL, CIGLR is at the forefront of implementing well-integrated observing systems that monitor key aspects of the Great Lakes environment. Data collected from buoys, autonomous underwater vehicles (AUVs), gliders, and satellites help us understand natural changes in the system, identify human-induced disturbances, support the development of climate, weather, and ecosystem forecasts, provide information for wise natural resource management, and fulfill critical information needs for public health protection and navigation safety.
Our Great Lakes observing activities include the:
1. Great Lakes Observing System (GLOS)
The GLOS network of buoys, autonomous underwater vehicles (AUVs), and gliders provides information on key weather, water conditions, hydrodynamic, and biological variables throughout all of the Great Lakes. GLOS focuses on four priority areas – climate change impacts, ecosystem and food web dynamics, protection of public health, and navigation safety and efficiency – to make real-time and historical data publicly available to the larger Great Lakes community. The information generated and distributed within the GLOS network is used to develop and improve Great Lakes models and forecasts, and provides recreational users with continuous wind and wave forecasts to enhance boater safety.
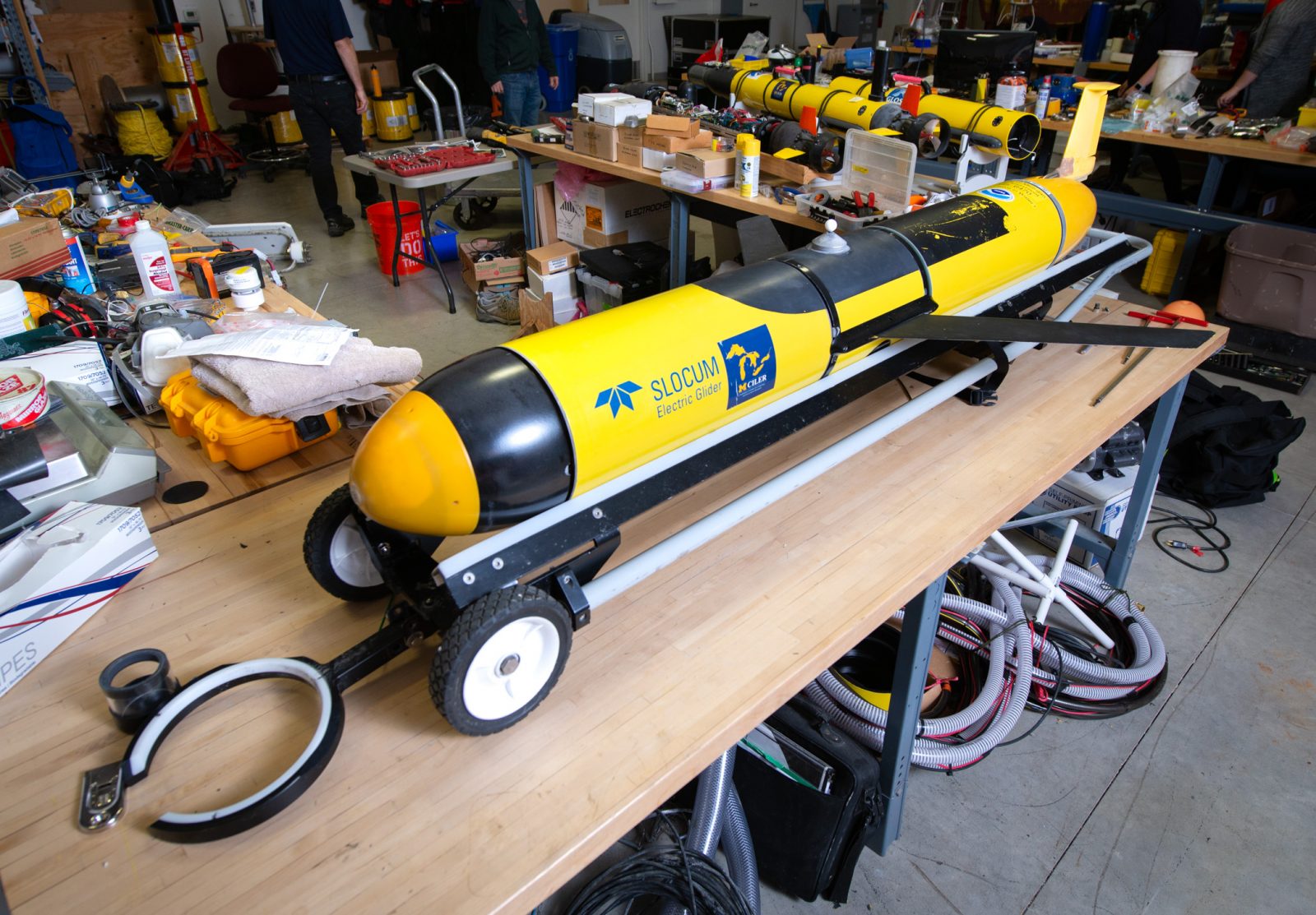
Data from buoys, AUVs, gliders, & satellites help us understand the critical information needs for public health protection & navigation safety. Click to see an interactive map of underwater glider missions. Photo Credit: Russ Miller.
CIGLR owns and operates two monitoring buoys in Lake Michigan and supports operation of a University of Michigan Biological Station buoy on Douglas Lake. These buoys are operational through the major boating seasons and report real-time water conditions, including data collection of high frequency wind, temperature and pressure data in support of ongoing meteotsunami research. CIGLR also owns and operates a Slocum glider, and two AUVs that supplement buoy observations by measuring conditions throughout the water column along defined paths in the lake. The gliders are able to traverse from one side of a Great Lake to another, and collect data from the surface to the deepest depths. Examples of AUV and glider applications include glider cross-lake surveys in Lake Michigan between Muskegon, MI and Milwaukee, WI and down to the National Data Buoy Center (NDBC) buoy in southern Lake Michigan to characterize thermal bar migration and the set-up of thermal stratification throughout the southern region of the lake; and in Lake Ontario, we worked with Consortium partners at Cornell University to conduct glider operations as part of the EPA led Coordinated Science and Monitoring Initiative (CSMI) to characterize spatial and temporal dynamics of the deep chlorophyll layer formation.
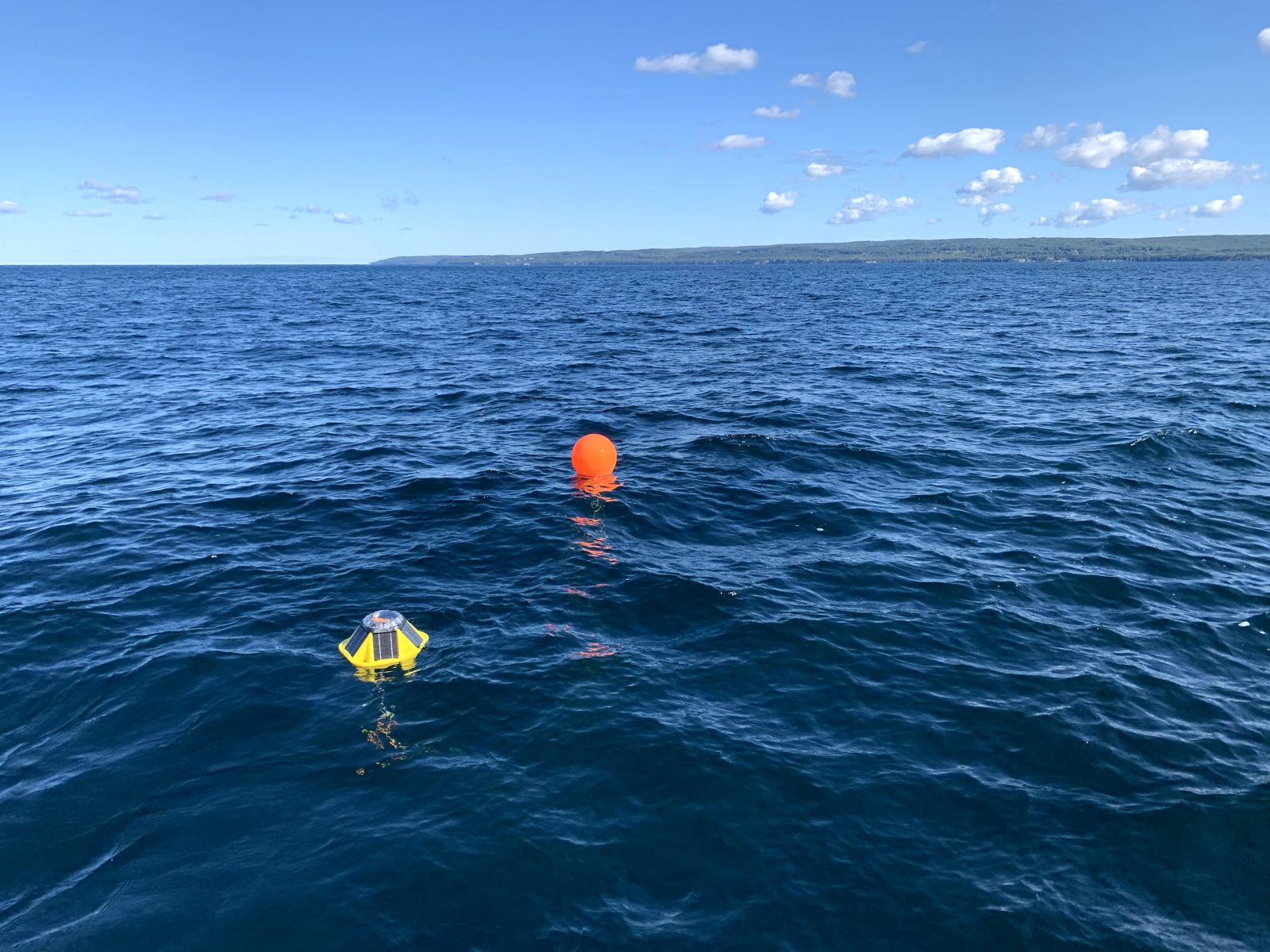
MTU and CIGLR stepped in to ensure buoys were deployed in Lake Superior despite delays due to COVID-19. Click image to read more.
When many larger research vessels were grounded due to COVID-19, CIGLR researchers were able to procure and deploy several smaller real-time wave buoys to maintain the flow of critical data for forecasters and Great Lakes stakeholders alike. These buoys, small enough to be lifted by hand, were deployed by small vessels at both new and long-standing GLOS buoy sites including Ludington, Little Traverse Bay, and multiple locations in Lake Superior (Michigan Technological University, Great Lakes Research Center). Building on the success of these deployments, these buoys will again be placed in new locations throughout the Great Lakes, leveraging stakeholder support for their deployment and recovery, and hopefully foster the start of a stakeholder supported program to increase coastal observations for research, recreation, and public/private safety.
Our stakeholder audiences are made up of a variety of public mission agencies that operate in a diverse range of disciplines, in multiple geographic locations, and in various organizations including: U.S. and Canadian federal government agencies; state, regional, local, and tribal government agencies; NGOs and partner initiatives; academic institutions; and private business, industries, and consultants. GLOS targets communications, outreach, and engagement towards those departments, agencies, and individual staff that can contribute to and/or benefit from GLOS programming, and that share common goals, objectives, and strategies with GLOS. This network includes formal and informal educators, modelers, and researchers who serve as target users in all of the GLOS focus areas.
2. Great Lakes CoastWatch
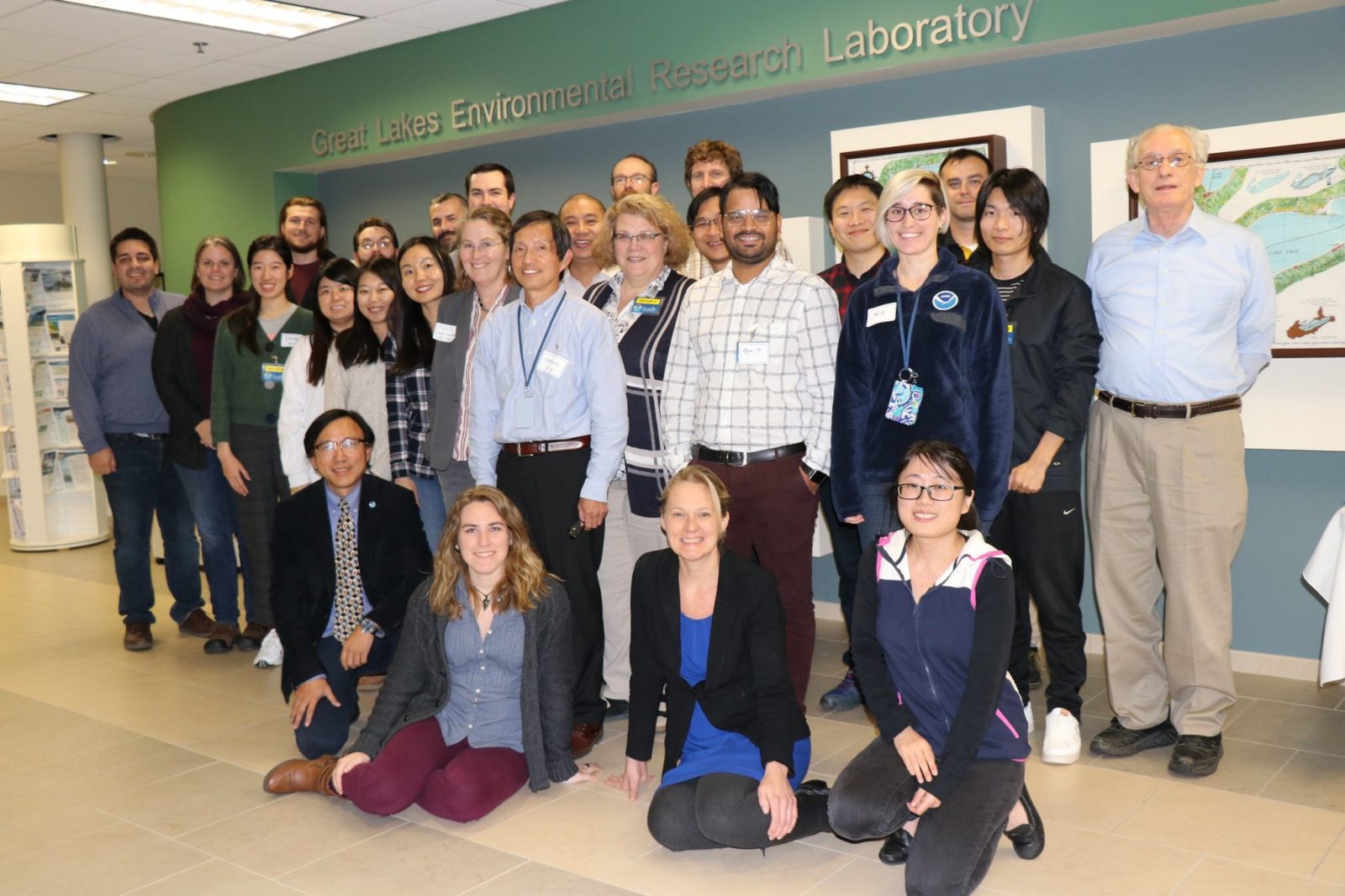
2019 CoastWatch Satellite Remote Sensing Course participants. Photo Credit: Katherine Glassner-Shwayder.
CoastWatch is a NOAA-wide program that provides continuous, near real-time, coordinated, environmental information (remotely sensed, chemical, biological, and physical) to support federal and state decision makers and researchers who are responsible for managing the nation’s coastal ecosystems. NOAA GLERL serves as the Great Lakes regional node of the nationwide NOAA CoastWatch program. CIGLR supports GLERL’s efforts to obtain, produce, and deliver environmental data products in support of research, management, and decision making in the Great Lakes and by producing satellite-derived maps, facilitating the dissemination of CoastWatch data and products, and researching algorithm development of remotely sensed data. The Great Lakes CoastWatch team provides access to near real-time and retrospective satellite and in-lake observations. Information and products from this program are used to help track algal blooms, pollution, ice cover, wind, and water intake temperatures at fish hatcheries; produce models of wave height, lake currents, and damage assessments; and support research, educational, and recreational activities. Users of this information include government agencies, such as the U.S. Coast Guard and National Weather Service, academic institutions, commercial interests, and the public.
3. Synthesis, Observations, & Response (SOAR) System
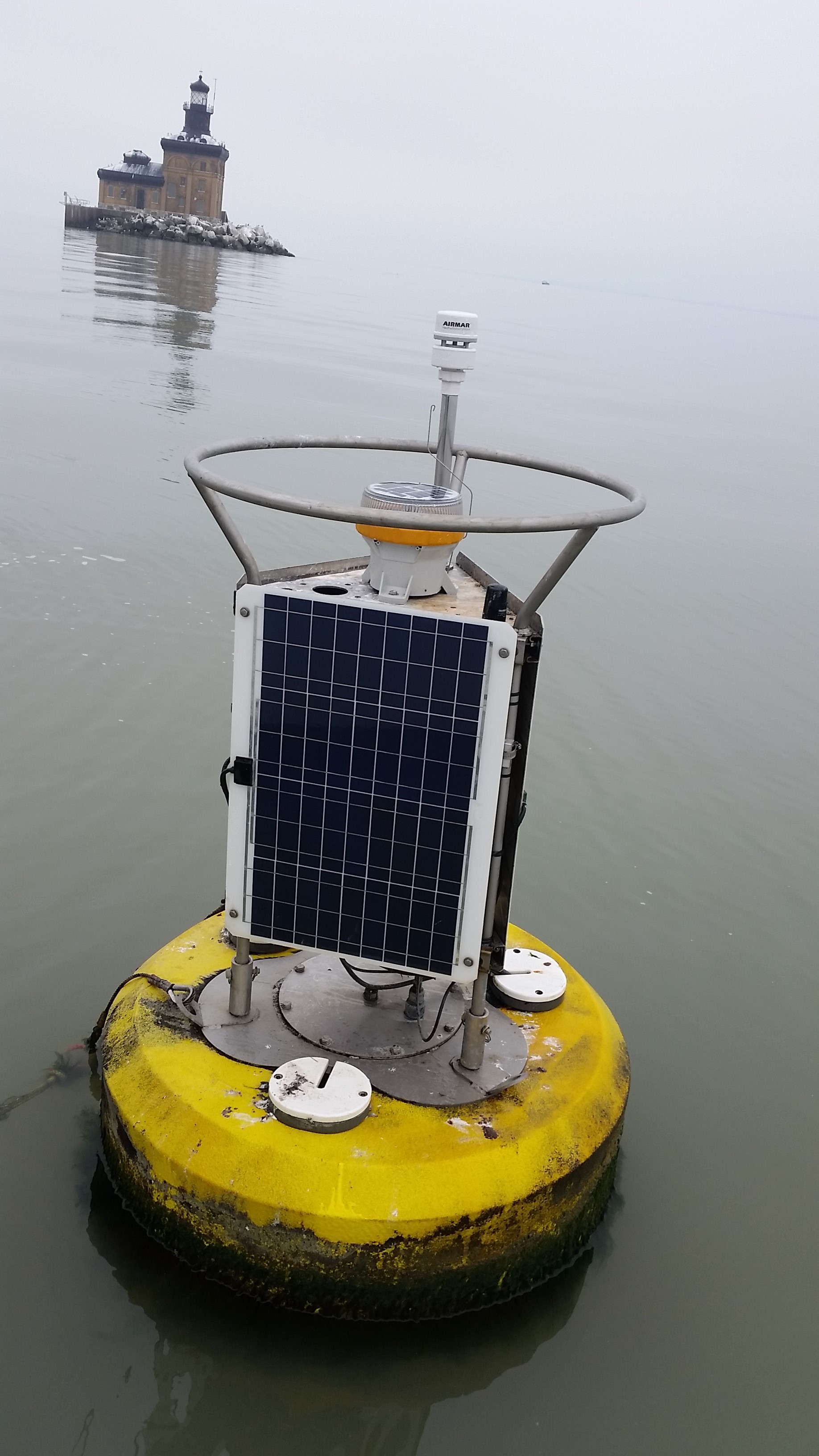
The SOAR Western Lake Erie buoy on a very calm day. Click image to learn more about SOAR. Photo Credit: Thomas Johengen.
The implementation of the Great Lakes Synthesis, Observations and Response System (SOAR) program is designed to coordinate and integrate regional coastal observations that support national and regional priorities, including Great Lakes restoration. SOAR activities include the deployment and support of on-water and remote sensing platforms, where observations from these systems are used to create database products for assessment and decision support through an up-to-date (including real-time data) web distribution. The SOAR system provides real-time ecosystem information to help public utility managers maintain high quality drinking water and informs the general public on current water quality conditions through observations, data management, and forecast model development. Observations of environmental parameters are used to develop decision support tools to provide warnings to regional managers regarding phosphorus loads, hypoxia and harmful algal blooms, and to support adaptive management process decisions. These decision support tools include: real-time observing systems (buoys) deployed at Maumee Bay, Saginaw Bay, Lake Michigan and Lake Erie; a web-based data management system; synthesized remote sensing products for predicting spatial extent, intensity, and duration of harmful algal blooms (HABs); and coupled physical-chemical-biological models for Saginaw Bay and western Lake Erie.
The overarching goal of the program is to improve our understanding of the causes of lake ecosystem impairments and contribute the necessary monitoring data to accurately track spatial and temporal trends in order to assess the effectiveness of remedial management actions. Program objectives include providing real-time data for web-based decision support systems for HABs and hypoxia, providing ground-truthing data for remote sensed estimates of HAB mapping and forecast information, generating satellite-derived HABs estimates for the Great Lakes, and collecting and processing inherent optical properties (IOP) data.
4. Cladophora / Submerged Aquatic Vegetation (SAV) Mapping
Piles of Cladophora algae have been washing up on Great Lakes beaches for generations. It became a nuisance in the mid-20th century, when unregulated nutrient runoff resulted in never-before-seen Cladophora blooms. Washing up in mats, the stagnant algae quickly started to smell, desecrated the shoreline and beaches, clogged water intakes, and created warm, nutrient-rich environments suitable for microbes, some of which proved harmful to humans and wildlife. By the 1980s, phosphorus reductions required by the Great Lakes Water Quality Agreement appeared to mitigate the Cladophora problem. However, fueled by the relentless invasion of zebra and quagga mussels and their impacts of increased water clarity and available phosphorus, the Cladophora problem is back, and Great Lakes scientists are working harder than ever to find a solution.
Michigan Tech Research Institute (MTRI) and partners including NOAA GLERL, CIGLR, the USGS Great Lakes Science Center, Environment and Climate Change Canada, and SUNY Buffalo State College are updating a 2010 MTRI satellite map that provided the first reputable estimate of Cladophora and other submerged aquatic vegetation (SAV) throughout the Great Lakes Basin. The 2010 Cladophora map was produced in cooperation with the EPA as part of the Great Lakes Restoration Initiative (GLRI) and has been used for a variety of applications including nutrient modeling, management decision-making, and predicting the outcome of possible new species invasions. Now, the team is working to make meaningful updates to this map, both to capture the changes over the past decade and to take advantage of new advances in lake bottom mapping and satellite platforms that better estimate the volume of Cladophora produced by the Great Lakes every year.
Overall, the new maps do not show a significant change in the percentage of the lake bottom colonized by Cladophora. They do, however, show that increases in water clarity across the lower four Great Lakes, which began in the mid-1990s in association with invasive mussels, have continued in many areas. Because clearer water allows algae to grow at greater depths while also allowing satellites to see through deeper water, both the percentage of the lake bottom that can be mapped and the total area of Cladophora beds are higher in the 2018 map relative to 2010. Results show a lot of variation among the Lakes; water clarity increased the most for Lake Huron, where the area of visible lake bottom increased by 29%, but actually decreased slightly for Lake Erie. As initially reported in 2017, Lakes Michigan and Huron are now even clearer than Lake Superior due to the predominance of mussels, as well as the effects of reduced nutrient loads.
This project demonstrates a successful approach to benthic mapping to automate much of the satellite image processing. In addition, the MTRI team are generating annual Cladophora maps for three “sentinel” locations in each Great Lake. The automation makes it possible to map large areas in much less time, while the ability to consume both Landsat and Sentinel imagery means that most areas of the Lakes can be mapped at least once every year. This work represents a significant development for satellite-based aquatic vegetation mapping and will provide resource managers further information on Cladophora growth hotspots and illustrate how the algae responds to different lake management tactics. The Environmental Protection Agency (EPA) and MTRI established a new goal to map the spatial extent of SAV eight years after the initial assessment to ascertain change. In addition, MTRI refined the mapping algorithm so that benthic, or lake bottom, features could be routinely monitored.
Stay up-to-date on the most recent news and scientific media generated from our Great Lakes Observing System Research research here:
Products & Resources
- Underwater Glider Missions (interactive map)
- Seeing Underwater with Coastal Gliders (factsheet)
- 2012-2019 Glider Deployment Catalog (data server – full data access)
- SOAR Project (factsheet)
- Great Lakes CoastWatch (website)
- GLERL Observing Systems and Advanced Technology (website)
- Muskegon Lake Buoy (website)
- Great Lakes Cladophora / SAV Mapping (website)
- Great Lakes Observing System (website)
- GLOS Great Lakes Buoy Portal (website)
- GLOS Data Portal (website)
News
- Where Modeling Meets Observations: Improving the Great Lakes Operational Forecast System, Michigan Tech News, 9/10/2020
- COVID-19 Delays Great Lakes Buoy Deployment, MTU Helps Fill the Gap, MTU News, 7/1/2020
- CIGLR Research Continues to Support NOAA’s Mission; Begins Phased Return to Critical Fieldwork, CIGLR 2020 Spring eNews, 06/2020
- Great Lakes Gliders Help Monitor Water Quality and Inform Hydrodynamic Models, CIGLR 2019 Fall eNews, 12/2019
- Great Lakes buoys help fight algal blooms, and they just returned to the water, Fox 2 Detroit, 5/24/2019
- GLOS News
Publications
Biddanda, B.A. 2017. Global significance of the changing carbon cycle. Eos- Earth and Space News, American Geophysical Union. 98(6):15-17. (DOI:10.1029/2017EO069751).
Bullerjahn, G.S., R.M. McKay, T.W. Davis, D.B. Baker, G.L. Boyer, L.V. D’Anglada, G.J. Doucette, J.C. Ho, E.G. Irwin, C.L. Kling, R.M. Kudela, R. Kurmayer, J.D. Ortiz, T.G. Otten, H.W. Paerl, B. Qin, B.L. Sohngen, R.P. Stumpf, P.M. Visser and S.W. Wilhelm. 2016. Global solutions for regional problems: collecting global expertise to address the problem of harmful algal blooms. A Lake Erie case study. Harmful Algae. 54:223-238. (DOI:10.1016/j.hal.2016.01.003). [Altmetric Score]
Biddanda, B.A., A.D. Weinke, S.T. Kendall, L.C. Gereaux, T.M. Holcomb, M.J. Snider, D.K. Dila, S.A. Long, C. VandenBerg, K. Knapp, D.J. Koopmans, K. Thompson, J.H. Vail, M.E. Ogdahl, Q. Liu, T.H. Johengen, E.J. Anderson and S.A. Ruberg. 2017. Chronicles of hypoxia: Time-series buoy observations reveal annually recurring seasonal basin-wide hypoxia in Muskegon Lake – A Great Lakes estuary. Journal of Great Lakes Research. (DOI:10.1016/j.jglr.2017.12.008). [Altmetric Score]
Carmichael, W.W. and G.L. Boyer. 2016. Health impacts from cyanobacteria harmful algae blooms: Implications for the North American Great Lakes. Harmful Algae. 54:194-212. (DOI:10.1016/j.hal.2016.02.002).
Cotner, J.B., A.D. Weinke and B.A. Biddanda. 2017. Great Lakes: Science can keep them great. Journal of Great Lakes Research. 43:916-919. (DOI:10.1016/j.jglr.2017.07.002). [Altmetric Score]
Defore, A.D., A. Weinke, M. Lindback and B. Biddanda. 2016. Year-round Measures of Planktonic Metabolism Reveal Net Autotrophy in Surface Waters of a Great Lakes Estuary. Aquatic Microbial Ecology. 77:139-153. (DOI:10.3354/ame01790). [Altmetric Score]
Fahnenstiel, M.J. Sayers, R.A. Shuchman, F. Yousef and S.A. Pothoven. 2016. Lake-wide phytoplankton production and abundance in the Upper Great Lakes: 2010-2013. Journal of Great Lakes Research. 42(3):619-629. (DOI:10.1016/j.jglr.2016.02.004). [Altmetric Score]
Kerfoot, W.C. and S.C. Savage. 2016. Multiple inducers in aquatic foodwebs: Counter-measures and vulnerability to exotics. Limnology and Oceanography. 61:382-406. (DOI:10.1002/lno.10223).
Kerfoot, W.C., N.R. Urban, C.P. McDonald, R. Rossmann and H. Zhang. 2016. Legacy mercury releases during copper mining near Lake Superior. Journal of Great Lakes Research. 42:50-61. (DOI:10.1016/j.jglr.2015.10.007). [Altmetric Score]
Kerfoot, W.C., M.M. Hobmeier, F. Yousef, B.M. Lafrancois, R.P. Maki and J.K. Hirsch. 2016. A plague of waterfleas (Bythotrephes): impacts on microcrustacean production in a large inland-lake complex. Biological Invasions. 18:1121-1145. (DOI:10.1007/s10530-015-1050-9).
Salk, K.R., P.H. Ostrom, B.A. Biddanda, A.D. Weinke, S.T. Kendall and N.E. Ostrom. 2016. Ecosystem metabolism and greenhouse gas production in a mesotrophic northern temperate lake experiencing seasonal hypoxia. Biogeochemistry. 131:303-319. (DOI:10.1007/s10533-016-0280-y). [Altmetric Score]
Sayers, M.J., A.G. Grimm, R.A. Shuchman, K.R. Bosse, G.L. Fahnenstiel, S.A. Ruberg and G.A. Leshkevich. 2019. Satellite monitoring of harmful algal blooms in the Western Basin of Lake Erie: A 20-year time-series. Journal of Great Lakes Research. 45:508-521. (DOI:10.1016/j.jglr.2019.01.005). [Altmetric Score]
Smith, J.P., R.J. Miller, R.W. Muzzi, S.A. Constant, K.Y. Beadle, D.A. Palladino, T.H. Johengen and S.A. Ruberg. 2017. An Implementation of a Database Management System for Real-Time Large-Lake Observations. Marine Technology Society. (DOI:10.4031/MTSJ.51.6.2).
Troy, C., D. Cannon, Q. Liao and H. Bootsma. 2016. Logarithmic velocity structure in the deep hypolimnetic waters of Lake Michigan. Journal of Geophysical Research: Oceans. 121:949-965. (DOI:10.1002/2014JC010506). [Altmetric Score]
Watson S.B., C. Miller, G. Arhonditsis, G.L. Boyer, W. Carmichael, M. Charlton, R. Confesor, D. C. Depew, T.O. Höök, S. Ludsin, G. Matisoff, S.P. McElmurry, M.W. Murray, P. Richards, Y. R. Rao, M. Steffen and S. Wilhelm. 2016. The re-eutrophication of Lake Erie: Harmful algal blooms and hypoxia. Harmful Algae. 56:44-66. (DOI:10.1016/j.hal.2016.04.010). [Altmetric Score]
Weinke, A.D. and B.A. Biddanda. 2017. From bacteria to fish: ecological consequences of seasonal hypoxia in a Great Lakes estuary. Ecosystems. (DOI:10.1007/s10021-017-0160-x). [Altmetric Score]
Xue, P., D.J. Schwab, R.W. Sawtell, M.J. Sayers, R.A. Shuchman and G.L. Fahnenstiel. 2017. “A particle-tracking technique for spatial and temporal interpolation of satellite images applied to Lake Superior chlorophyll measurements.” Journal of Great Lakes Research. 43(3):1-13. (DOI:10.1016/j.jglr.2017.03.012). [Altmetric Score]
PrincipaI Investigator(s):
Russ Miller (CIGLR)
NOAA Technical Lead(s):
Steven Ruberg (NOAA GLERL)
Andrea Vander Woude (NOAA GLERL)
GLOS Photo Gallery
CIGLR Engineers Russ Miller and Heidi Purcell deploy the NOAA GLERL western Lake Erie buoy in July 2020. Buoys are a great source of Great Lakes data. They provide measurements like wind speed, water and air temperature, and wave height. Photo Credit: Kent Baker.
A beautiful day to be on the water caring for our Great Lakes buoys during 2020 field season. Photo Credit: Hayden Henderson.
Data from buoys, AUVs, gliders, & satellites help us understand the critical information needs for public health protection & navigation safety. Photo Credit: Russ Miller.
2019 CoastWatch Satellite Remote Sensing Course participants. Photo Credit: Katherine Glassner-Shwayder.
Buoy tune-up & deployment on Lake Erie! We use data from these systems to create forecasting products that evaluate restoration effectiveness, provide ecosystem assessments, and aid in decision support for regional managers. Photo Credit: Russ Miller.
Russ Miller and Tom Johengen are servicing one of the four western Lake Erie real-time water quality monitoring buoys that have been developed to support the SOAR and HABs projects at CIGLR and GLERL. Once a month they service the moorings by replacing, or cleaning and recalibrating, the water quality instruments which include the WetLABS Cycle P for measuring soluble reactive phosphorus, the Satlantic SUNA for measuring dissolved nitrate, and the YSI EXO2 multi-parameter sonde which measures temperature, conductivity, pH, DO, Chlorophyll, Phycocyanin, and turbidity. Summer 2016. Photo Credit: Heather Miller.
Russ Miller and Tom Johengen are servicing one of the four western Lake Erie real-time water quality monitoring buoys that have been developed to support the SOAR and HABs projects at CIGLR and GLERL. Once a month they service the moorings by replacing, or cleaning and recalibrating, the water quality instruments which include the WetLABS Cycle P for measuring soluble reactive phosphorus, the Satlantic SUNA for measuring dissolved nitrate, and the YSI EXO2 multi-parameter sonde which measures temperature, conductivity, pH, DO, Chlorophyll, Phycocyanin, and turbidity. Summer 2016. Photo Credit: Heather Miller.
Russ Miller and Tom Johengen are servicing one of the four western Lake Erie real-time water quality monitoring buoys that have been developed to support the SOAR and HABs projects at CIGLR and GLERL. Once a month they service the moorings by replacing, or cleaning and recalibrating, the water quality instruments which include the WetLABS Cycle P for measuring soluble reactive phosphorus, the Satlantic SUNA for measuring dissolved nitrate, and the YSI EXO2 multi-parameter sonde which measures temperature, conductivity, pH, DO, Chlorophyll, Phycocyanin, and turbidity. Summer 2016. Photo Credit: Heather Miller.
Tom Johengen services one of the four western Lake Erie real-time water quality monitoring buoys that have been developed to support the SOAR and HABs projects at CIGLR and GLERL. Once a month they service the moorings by replacing, or cleaning and recalibrating, the water quality instruments which include the WetLABS Cycle P for measuring soluble reactive phosphorus, the Satlantic SUNA for measuring dissolved nitrate, and the YSI EXO2 multi-parameter sonde which measures temperature, conductivity, pH, DO, Chlorophyll, Phycocyanin, and turbidity. Summer 2016. Photo Credit: Heather Miller.
Video Library
Researchers use robotic underwater gliders that travel for miles to collect data on organic matter, conductivity, oxygen content, and more, sometimes staying out for three months! Watch as Russ and Hayden “unbox” the glider and explain what this amazing device is capable of.
fdgj
Lauren Marshall worked with CIGLR as a 2019 Great Lakes Summer Fellow. She processed environmental data collected by autonomous underwater vehicles (AUVs) in the Great Lakes and helped to visualize the data so that it can be used to engage the public and stakeholders.
.
fdgj
Anisha Shrestha worked with CIGLR as a 2020 Great Lakes Summer Fellow. She worked to identify patterns in Great Lakes environmental data collected by an underwater glider.
.
.
fdgj
Technicians get a brand new underwater glider ready for deployment, testing the fins, propeller and more. These underwater gliders “fly” through the water slowly to take measurements on water health and environmental conditions.